Seismic
Sequence Analysis
Exercise Four - Introduction to the Stratigraphy of the Neogene
of the Western Bahamas

This
is the fourth of the exercises tied to the interpretation of
seismic data and unlike the previous two exercises concentrates
on the seismic response of carbonate sediments.
INTRODUCTION
The objective of this exercise is to interpret the sequence
stratigraphy of the Neogene carbonate section displayed on a
seismic line across acquired by Western Geophysical on the northwest
corner of the Great Bahamas Bank, just west of the coast of
Andros Island (Eberli and Ginsburg, 1987). The geological setting
of the exercise is the Great Bahamas Bank, which overlies part
of the edge of the North American continental crust and the
adjacent oceanic crust of the Western Atlantic (Pindell 1985).
The Great Bahamas Bank was initiated by the accumulation of
a mix of siliciclastic, carbonates and evaporites in the Late
Triassic and Early Jurassic but most of the sedimentary fill
is from the Jurassic to Holocene is composed of carbonate sediments
with the thickest sections occuring in the Cretaceous and Tertiary
(Eberli and Ginsburg, 1987).
The
exercise concentrates on the study of the geometric relationship
of carbonate sequences expected in the shelf, reef crest, slope
and down slope basin floor fans to local changes in base level.
In the Bahamas these changes in base level were probably driven
by a combination of tectonic movement and the Neogene eustatic
signal preserved in the stratigraphic record of these carbonate
rocks.
THE
PRE HOLOCENE SEDIMENTARY SECTION OF THE GREAT BAHAMAS BANK
The inference
from seismic, gravity, magnetic, and well data is that the northwest
Bahamian platform is on the edge of the North American continental
crust, while to the southwest it is situated over oceanic crust
(Pindell, 1985). This is supported by seismic and magnetic data
which indicates that the approximate depth to the source of
magnetization in the southeastern Bahamas is at about 10 km
(Bracey, 1968; Drake et al., 1963; Sheridan et al, 1966; Talwani,
1960; Uchupi et al., 1971; Pindell, 1985). Similarly, gravity
data indicates that the depth to the mantle is about 12 km beneath
the Atlantic abyssal plain adjacent to the platform; it is about
20 km beneath the southeastern Bahama platform; and about 30
km beneath the northern Bahama platform (Houtz & Ewing,
1964; Talwani, 1960, Uchupi et al., 1971). In the southeastern
Bahamas, where the crust is only about 10 km thick, though most
of it is made up of carbonates it is much too thin to be considered
normal continental crust. In contrast, to the northwest, the
section interpreted as sedimentary approaches continental thickness.
Similarly regional seismic profiles suggest that the depth to
oceanic crust increases from the adjacent abyssal plain toward
the edge of the Bahama escarpment (Ball et al., 1971; Uchupi
et al., 1971).
Parallel
to, and east of the present Bahama Escarpment, seismic, magnetic
and gravity data suggest the presence of a deeply buried scarp
feature in the oceanic crust. Uchupi et al (1971) point out
that the trend of the buried scarp, which they interpret as
related to a fracture zone, is exactly on a small circle for
spreading in the north Atlantic, with the pole of rotation at
65oN, 12.5oW. Magnetic anomaly patterns to the east are perpendicular
to the fracture, suggesting the possibility of a ridge-ridge
fracture pattern as the possible origin of this feature. This
idea is enhanced by the lack of fit for the platform in a sea
floor spreading reconstruction of the Atlantic (Dietz et al.,
1970). Based on a modified Bullard reconstruction of the closure
of plate boundaries to before the development of the Atlantic
Ocean (Bullard et al., 1965; Dietz et al., 1970), virtually
all of the Bahamas overlap onto the African continent and its
continental shelf. Deitz et al., (1970), Uchupi et al., (1971),
Le Pichon and Fox (1971), Glockhoff (1973), and Sheridan (1974
a&b) all subscribe to the theory that the Bahama platform
developed over oceanic crust, and that this development occurred
during the earliest phase rifting and sea floor spreading that
eventually created the Atlantic. Pindell (1985), in contrast,
is of the opinion that much of the Bahamas is underlain by attenuated
and intruded pre-Mesozoic crust. He includes the western half
of the Bahamas in a plate he calls the Florida Straits Block.
It is because in plate reconstructions with the present Atlantic
closed the crust of the Florida Straits block overlaps Africa
that Pindell (1985) proposes that this plate must have migrated
from a position in the eastern Gulf of Mexico. During the Jurassic,
asymmetric rifting separated North America from Europe, Africa
and South America forming a narrow isolated basin that could
have been similar to the present Red Sea. A comparable model
would be the Gulf of Mexico or possibly the Mediterranean.
Gravity
models indicate evaporites (or similar material) are included
in the sediment stack and account for the gravity values for
the region. This basin nearly filled to sea level over a period
of 15-20 million years. Carbonate sedimentation began on localized
basement highs, and perhaps over shallow portions of the oceanic
ridge beneath the southeastern Bahamas (Uchupi et al., 1971).
Like Pindell (1985), Meyerhoff and Hatten (1974 a&b) argue
that the Bahamas are underlain by continental crust. Mullins
and Lynts (1977) agree and suggest any crustal overlap in plate
reconstructions can be taken care of by rotating the Bahamas
area 25 degrees to the northeast during Cretaceous or early
Tertiary time, in response to motion of the Caribbean plate.
Once initiated, the production of shallow water carbonates at
the surfaces of the shallow platforms was able to keep pace
with continued subsidence of the oceanic crust and the ever-increasing
weight of the carbonate section. Following the initial period
of asymmetrical crustal attenuation, shearing and continental
basin filling in the Late Triassic, symmetrical oceanic spreading
then began in mid Jurassic time, about 170 MY BP (Pindell, 1985).
This carried the Bahama platform westward on the trailing edge
of the North American plate, and completing development of the
ridge-ridge transform fault along the eastern margin of the
complex.
Several
features that might be expected in the structure of a carbonate
platform are seen in a seismic refraction profile made by the
University of Miami (Ball et al., 1971). This lies seaward of
Cat Island on the eastern side of the Great Bahama Bank and
seaward of Exuma Sound. It exhibits travel times at depths that
are consistent with what should be expected of a thick carbonate
platform . Lithologic interpretations coupled to travel time
velocities (from seismic refraction profiles) were: 2.0-2.5
km/sec = unconsolidated sediments; 4.7 km/sec = consolidated
Cenozoic to Upper Cretaceous carbonates; 6.1-6.5 km/sec = consolidated
Mesozoic carbonates and basement rocks; 8.1-8.3 km/sec = upper
mantle. These data suggest that beneath the Bahama platform,
the mantle plunges abruptly to near continental depths. Also,
the inferred top of the Lower Cretaceous is clearly depressed
beneath Exuma Sound, indicating structural down-drop within
the basin.
Several
reflection profile studies have revealed folding and faulting
of the sediments of the Old Bahama Channel, adjacent to Cuba
(Sheridan et al, 1966; Uchupi et al, 1971). Pindell (1985) records
that the southern margin of the Bahamas was imbricated in the
Paleogene into thrusts when Cuba collided with it. This may
be correlated with shallow seismic activity along the trend
of Cuba. Not only that but reflection profiles also reveal folded
and faulted sediments in the Tongue-of-the-Ocean, Exuma Sound,
and Columbus Sound (Ball et al., 1971). The faults may displace
the present sea floor, and suggest sedimentation rates two to
three times less than the up-thrown platforms. Clearly these
faults are active at the present time. Combined with the negative
Bouguer gravity anomalies for re-entrant areas (Talwani, 1960),
these observations indicate a basement structural control on
the position of the deep re-entrants; moreover, down-drop along
these features is still going on.
SEDMENTARY
HISTORY
The sedimentary history of the Bahamas from Late Triassic times
is determined from a combination of seismic profiles and wells.
Only a few deep well tests have been drilled within the Bahama
area (Andros 1, Long island 1, Great Isaac 1, and Cay Sal IV-1)
to the Jurassic (Meyerhoff and Hatten, 1974 a&b), while
a number of shallow water wells have been drilled by the Bahamian
Government and three by the University of Miami (Beach and Ginsburg,
1980). Off platform, a series of wells have been drilled and
cored by the Deep Sea Drilling Project and the Ocean Drilling
Program (Hollister, Ewing et al., 1972, and DSDP Scientific
Party, 1985). Deep dredgings have collected limestone from the
bank margin (Freeman-Lynde, 1981; Corso, 1983; Schlager et al.,
1984; and Freeman-Lynde and Ryan, 1985). Much of these data
suggest the Mesozoic sediments of the Bahamas are largely shallow
water carbonates with some evaporites. The well drilled on Cay
Sal Bank to the west of the Great Bahama Bank bottomed in Upper
Jurassic shallow water carbonates at 5700 meters. The well at
Great Isaac passes through Jurassic shallow water carbonates
and black shales and penetrates Triassic arkosic volcano clastics,
the oldest section seen in any of the wells (Tator & Hatfield,
1975). The well at Long Island bottomed in Upper Jurassic shallow
water carbonates at about the same depth as Deep Sea Drilling
Project site 100, which was drilled in the abyssal plain just
to the east. DSDP cored the contact between the sedimentary
section and underlying oceanic crust. Here, late Jurassic (approximately
160 Ma) Oxfordian argillaceous carbonates immediately overlie
basaltic pillow lavas (Spencer, 1967). Probably, the best studied
published core data comes from the Andros Island well Number
1, which terminated in Lower Cretaceous shallow water carbonates
with a T.D. of 4446 meters (Spencer 1967, and Goodell and Garman,
1969). The uppermost 15-20 meters of the Andros cores consist
of oolitic sediments while the rest is composed almost entirely
of pellet muds similar to those accumulating on the present
platform interior. Mineralogically, the cores show a series
of gradations of limestone to thick dolomite. For instance,
sediments are 100% limestone in the upper 20 meters of the section,
and increase in dolomite content downward, with 100% dolomite
being reached at 120 meters. Four such sequences of thin limestone
and thick dolomite units are found in the cores. The dolomitization
began initially as a micro-crystalline replacement fabric (replacing
both matrix and pellets). Crystal size in the dolomite units
seems to increase downward. Where solution of microfossils has
occurred, coarser dolomite spar fills fossil molds. Fractures
are quite common in the section, and are often filled with coarse
dolomite spar (Goodel and Garman, 1969).
Relative
to other carbonate regions there is less published on the generation
of the various fabrics and mineralogies in the Bahamian cores.
Using sections in Cuba, Goodell and Garman (1969) correlate
the top of the limestone units with periods of orogeny and/or
exposure. These authors account for the dolomitization through
the "cannibalization" of magnesium from the surficial
limestones, together with downward reflux of Mg-rich brines.
Other hypotheses, including dolomitization in a mixing zone
between fresh and salt water are equally plausible.
McNeill
and Ginsburg (1988) have utilized magnetostratigraphic dating
to determine the age of Cenozoic carbonates and their rates
of accumulation. They re-examined old core data from San Salvador
Island and determined the depth of magnetic reversals in the
late Cenozoic shallow-water carbonates which they then compared
to data from two cores taken on Andros and Williams Islands.
These results have provided new data and dates that could revolutionize
our concepts of bank development. Their cores record the upper
section of the Gauss chron, the reversed Matuyama, and the normal
Brunhes. The late Eocene section, which was previously dated
with only a generalized biostratigraphic age, is now dated at
between 36.5 and 37.5 Ma (chron 15 and 13r).
The
magnetic minerals they found in the Bahamian carbonates are
thought to be biogenic in origin because of their similarity
in size (0.04-0.3 µm), composition (magnetite), and crystal
morphology to biogenic magnetite. McNeill and Ginsburg (1988)
have applied this approach to recrystallized and dolomitized
carbonates, providing a new means for more precise dating of
the changes in the late Pliocene Bahamian reef-rimmed atoll
to a flat-topped bank. They were able to date the last occurence
of numerous mollusks and corals. They plan to determine rates
of sediment accumulation in different bank settings as more
core data becomes available.
Another
possible use of this method is to collect samples from the extensive
cave systems that exist in the Bahamas. The McNeill and Ginsburg
(1988) data indicate that the Pleistocene/Pliocene contact should
be at about 42 m (135 ft) on Andros Island, a depth easily attainable
by scuba diving. Off San Salvador they put the Pliocene/Miocene
boundary at about 115 m (380 ft), a depth that the small submersibles
used by the CMRC can reach. It is interesting to note that the
115 m (380 ft.) depth level corresponds to the depth at which
karstification and caves have been observed off Lee Stocking
Island, Long Island in Exuma Sound, and New Providence Island
in the Tongue-of-the-Ocean. We hope this magnetostatigraphic
dating technique can be used for precisely oriented and located
samples from Normans Pond Cay Cave.
Eberli
and Ginsburg (1987) have published and interpreted a series
of seismic lines for the northwestern portion of the Bahamas
Bank. They suggest these lines indicate that in the Late Cretaceous/Early
Tertiary the northwest Great Bahama Bank was segmented into
three smaller platforms that were separated by re-entrants that
later were filled by bank top and slope sediment that prograded
from east to west. Interestingly, the western margins were smothered
by abundant sediment with an eastward source while they interpret
the eastern margins of the platforms as the site of reef growth.
A relationship to the prevailing easterly winds and a high-energy
exposed reef encrusted margin is indicated. Sediment produced
by a growing reef system and to its lee would presumably have
been carried to the leeward margin of the bank to create this
prograded leeward margin. However the greatest proportion of
the leeward prograding sediments were probably composed of peripelagic
oozes and turbidites. Eberli and Ginsburg (1987) relate the
origin of the now buried re-entrants to a combination of block
faulting coupled to climatic and eustatic stress which temporarily
turned off or slowed carbonate production, initially starving
the graben of sediment. Though no seismic lines for the Mid-Jurassic,
Early and Mid-Cretaceous have been published, it seems likely
that the pattern of re-entrant and bank morphology recognized
by Eberli and Ginsburg (1987) for the Late Cretaceous and Early
Tertiary probably existed then too. This pattern of bank segmentation,
starvation, progradation, and fill is supported by Leg 101 ODP
results (Leg 101 Scientific Drilling Party, 1985 a & b)
arguing against the hypothesis that the Bahamas have remained
a single megabank through their history.
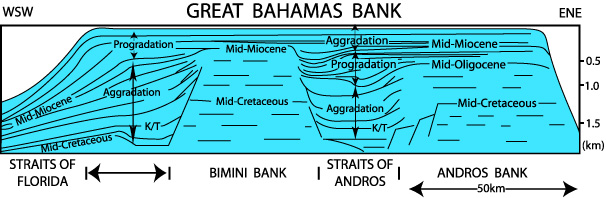
It is important to realize that a tremendous amount of sediment
must have been produced in shallow water to fill these ancient
re-entrants and that submarine processes were extremely active
in removing bank top production into deeper water. It is also
important to note that this is a period of time when the seas
were extremely productive. The closing of the deep water connection
of the Atlantic and Pacific through Central America in the early
Miocene caused the northward deflection of the North Atlantic
Equatorial Current and intensified the Gulf Stream (Keller and
Barron, 1983). It probably also increased the upwelling of carbonate
charged waters along the eastern margin of the Bahama limestone
platform as well and thus increased their ability to support
a larger carbonate producing community of organisms. This could
help explain why there was a dramatic increase in productivity
and the resulting filling of the ancient re-entrants during
the Middle Miocene. The period when prograding seems to be most
active, was a time when extensive beds of phosphorite were also
deposited along the continental margin of the east coast of
the U.S., in particular in northern Florida (Riggs, 1984) indicating
a local increase in the nutrient content of the waters. This
was the time when the largest shark ever (over 20 m-long, Carcaridon
megalodon ) swam Miocene waters throughout the Tethys seas.
To maintain its size at the top of the food chain, this animal
must have had a prodigious appetite that would have required
a highly productive source of food. The extensive and thick
deposits of shallow water Middle Miocene limestones that were
deposited on the continental margins of all continental land
masses confirm this high carbonate productivity and may help
explain how the deep re-entrants could be fill in a relatively
short period of geologic time. Eberli and Ginsburg (1987) have
related the series of prograding off lapping beds seen at a
two-way travel time depth of 0.6 km/sec on the seismic profile
shown in Fig. 8 to the fluctuations in sea level, described
by Haq et al., (1987) and Vail, et al. (1977).
In view of the Bahamas' checkered and complex geologic history,
the most striking aspect of this carbonate complex is its vertical
scale. Both the thickness in section of shallow water and prograding
slope carbonates continually deposited from Jurassic, and the
depth differential between the platforms and the empty and/or
filled oceanic re-entrant deeps are greater than any other carbonate
platform known from the geologic record. Comparison with Atlantic
abyssal plain sediments just east of the platform complex suggests
that preferential subsidence has occurred under the platform.
This may be a function of the location of the platform complex
at a continent/oceanic crust margin, and perhaps only in this
situation is the potential for such massive crustal subsidence
and carbonate progradation found. The Bahamian platform does
have one unique feature in that it is also bounded by the Puerto
Rican Trench, an area of subduction further to the south. It
is speculated that the transition of the Northern Puerto Rico
trench from a region of subduction to one of strike slip movement
was due to the inability of the trench to "swallow"
the lighter carbonates in a subduction zone (Ref.). The massive
thrust sheets on north Cuba support this contention. In short
the carbonates refused to be "flushed-down" the trench's
throat.
However
unique the Bahamian platforms may appear relative to their thickness,
there are many examples of ancient carbonate platforms with
the same general construction, i.e., shallow flat-topped platforms
separated by steep-sided narrow basins or re- entrants that
are filled by the local progradation described by Eberli and
Ginsburg (1986). Examples include the upper Devonian of the
Canning Basin, Western Australia (Playford, 1980), the upper
Devonian of Western Canada (Stoakes,1980), the Permian of western
Texas (Newell et al.,), the Upper Jurassic and Lower Cretaceous
of the Neuquen Basin in Argentina (Mitchum and Uliana1988),
the Mesozoic of the Dolomites (Bosellini et al.,1980, Bossilini
1988) and the Appenines (D'Argenio et al 1975). The same type
of structural control on basin and platform configuration can
be postulated, although the underlying basement is likely to
have been different. Thus, the same principles of sediment distribution,
the processes of formation, environmental and geochemical constraints
and oceanographic mechanisms of geological formation should
be as applicable to ancient platforms as they are to the Bahama
complex. It is this supposition "that the general principles
of carbonate sedimentation" have prevailed through geological
time that permits using the Bahamian sedimentary relationships
as a comparative model for Holocene and older shallow marine
carbonate deposition. The paleoceanographic conditions would
still depend on the circulations of the winds and the configurations
of the surrounding land masses, it is thus the "fun"
part of any comparative study to try and determine these relationships
and the constraints one must put on developing the model.