Summary
The varied depositional settings in which carbonate accumulate have become better known largely through the exploration for more hydrocarbon reserves and their exploitation in the last 35 years. Though reefs and grainstone shoals have been cited as common hydrocarbon exploration targets and so have been more intensively examined, other carbonate facies from both basin and/or platform interior settings have also been recognized to be important and have been studied too.
Though reefs and grainstone shoals have been cited as common hydrocarbon exploration targets and been more intensively examined, other carbonate facies from both basin and/or platform interior settings have also been recognized to be important and have been studied to
Collectively studies have shown that carbonate facies are commonly the product of processes that are active in their depositional setting. Water depth, winds, waves, currents, temperature, water chemistry, and biologic action all affect the character of the carbonate formed. Diagenesis, which also plays a major role in forming and modifying the facies that are found in the subsurface, are dealt with separately in another section of this web site. Below we show that it is possible to group carbonate facies on the basis of their depositional settings (table below and figure above) and their response to changes in base level (often sea level).
These depositional settings can occur along continental margins, within epeiric seas, or on isolated platforms in oceanic basins (figure above).
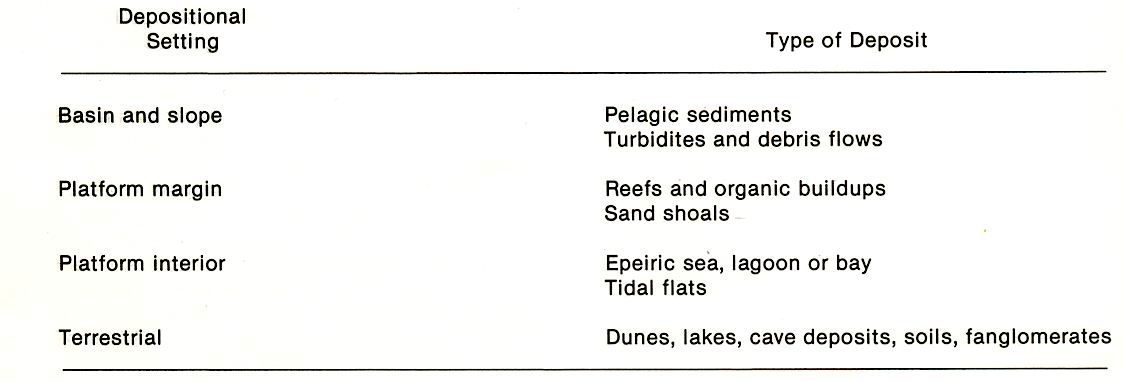
High and low frequency changes in base level are believed to produce the vertical cyclic character exhibited by the sedimentary facies associated with of these carbonate settings and their common interface with terrigenous systems. It has been recognized that sediment layering and the surfaces that bound these layers have predictable continuity. This layering has been related to particular base level positions and has enabled a better understanding of the lateral and vertical relationships of carbonate sedimentary facies. This has been used to build better hydrocarbon exploration and production models for the petroleum industry and is the reason that we have the understanding of sediment facies and their geometries that is summarized below.
BASIN AND SLOPE
Pelagic Sediments
Pelagic calcareous sediments are open marine deposits that often form close to the water surface in the photic zone but collect below depths affected by wave action. They usually found at the seaward margins of shelves and platforms and extend basinward into areas below the photic zone. The percentage of pelagic carbonates in deep-sea sediments varies as a function of depth, because with increasing water depth there is increasing dissolution of aragonite and calcite skeletal particles. In modern oceans aragonite tends to dissolve at depths between 500 m and 1500 m, while calcite tends to dissolve at depths that are substantially deeper and between 750 m and 4300 m. The end result is that at great depths or in cold-water areas calcite skeletal components completely dominate calcareous oozes. In modern oceans at depths below about 4300 m, all carbonate particles dissolve and only fine terrigenous sediment is observed. Terminology used to describe these effects include the Lysocline is the depth at which dissolution of calcium carbonate is manifest and is shallower than Calcium Carbonate Compensation Depth (CCD) which is the depth at which ocean floor sediments have less than 10% Calcium Carbonate. Both tend to be in shallower water in the higher latitudes and become deeper towards the equator.
Development of nodular carbonate in deeper water
carbonate<>
Bedding in open marine deposits is usually thin (5-10mm., rarely much thicker than 100 m). The characteristically thin beds are the result of the dominant mechanism of deposition, gravitational settling. Bed surfaces are planar to nodular (left figure) and sets of beds may be continuous over wide areas. Uneven nodular fabric, typical of many deep-sea carbonates, may originate in a number of ways. The nodules may be the result of burrowing or may also be produced by some initial marine cementation followed by pressure solution during burial. Because these sediments are deposited very slowly, they may be cemented by calcium carbonate, are commonly mineralized and may be encased by crusts of hematite, glauconite, pyrite and manganese.
Changes in base level lead to cyclic variations in the vertical character and composition of basinal sediments. In deeper water away from the platform, during high stands the sediment tends to be a mixture of pelagic tests, lime mud which may be interbedded with lowstand carbonates, and/or often silciclastic sediments, usually marl or shale but sometimes thin sands or siltstones. Toward the basin margin the highstand sediment becomes a mixture of pelagic and benthonic skeletal hash in a matrix of lime mud which are in turn interbedded with lowstand sediments often silciclastic sediments, commonly marls or shale, but including thin sands or siltstones. In both deep and basin margin settings the carbonate mud sized fraction predominantly consists of calcareous nannoplankton, broken pieces of planktonic foraminiferal tests, and fine sediment that is winnowed from adjacent shallow platforms and has settled through the water column. Pre-Jurassic deep-sea limestones are the most difficult to interpret because of the apparent worldwide absence of calcareous nannoplankton and planktonic foraminifera. The source of this carbonate is not fully understood. These deposits are usually interbedded with shales and marls and are dark in color. This shale and/or marl is commonly interpreted to be a response to lowstand deposition.
Source and Reservoir Potential
Although basinal sediments are dark colored and give off a fetid odor upon fracture and so are generally regarded as potential source rocks, they frequently contain little organic carbon. Thus, most deep-sea carbonates are not significant hydrocarbon sources for shallow shelf carbonate traps. However high organic matter production and euxinic conditions are more likely to prevail in shallower basins that have become isolated within the cratonic interiors or margins, or during the initial pull apart of continents. It is within these basins that source rock potential can be high. This was particularly true of the Arabian Gulf area where local intercratonic basins contain the basinal source rocks from the Middle Jurassic (Hanifa Formation) and the Lower Cretaceous (Lafan Formation) and supplied for many of the giant fields of the area.
Deep-sea micritic limestones generally have very low porosities and permeabilities, but are susceptible to fracturing and so form significant reservoirs at a number of localities. Primary porosity in pelagic chalks may be extremely high (e. g., 650/0 in Miocene chalks of the Caribbean), but this porosity is largely due to open chambers of pelagic foraminiferal tests and a loosely packed nannofossil matrix, thus their permeability is low. Chalks are significant reservoirs in the upper Cretaceous and Tertiary of the North Sea and the Upper Cretaceous of the U. S. Gulf Coast (the Austin Chalk Trend). North Sea chalks produce from a combination of preserved primary porosity, moldic porosity and fracture porosity; whereas the Austin Chalk produces where fractured.
Turbidites and Debris Flows
Basinal sediments shed from shelf margins include turbidites and debris flows. Turbidites are sediments transported and redeposited by turbidity currents. These deposits exhibit similar sedimentary structures whether they are carbonate or siliciclastic turbidites (figure below). Sediment type within carbonate turbidites is variable and controlled by source. Turbidites are among the few carbonates that have been transported significant distances. Close to the basin-platform margin carbonate turbidite deposits form irregular ribbons of channel-fill that are perpendicular to depositional strike. Farther from the margin, the turbidites form fan-shaped wedges which in cross-section are composed of evenly-bedded sheets. Bedding varies from thin to almost massive. These sediments, transported from shallow platform and upper slope, may include reef debris, oolites, lime mud or broken limestone blocks. Earthquakes, sea level changes or slope failure may initiate transportation.
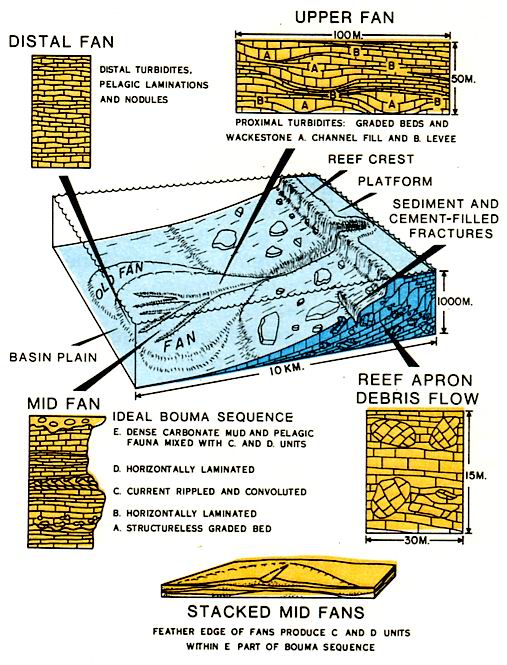
Carbonates deposited at margin between shallow-water platform and deeper water basin
Proximal carbonate turbidites are characterized by the lower part of the Bouma graded bed sequence and massive wackestones in which a lime mud matrix supports the grains. The basal layers of turbidites may be unsorted and may include ripups from the substrate. Bedding is irregular and discontinuous because the sediment is deposited as channel fills and levees on submarine fans. Bases of beds fill over match the contours over the scoured upper surfaces of previously deposited turbidites. Sediment composition and grain size are extremely variable and may include a wide range of shallow water biota mixed with pelagic skeletons. Distal turbidites are characterized by the upper part of the Bouma graded bed sequence. Bedding is regular, planar, and commonly the turbidites are interbedded with marls. These turbidite sediments are deposited at the margins of submarine fans some distance from their source. The biota is much the same as that of proximal turbidites except that there is a greater percentage and diversity of pelagic skeletal debris.
Carbonate debris flow deposits are characterized by a mixture of a wide variety of lithologic types and sizes of angular fragments that have anomalously oriented stratification. They include megabreccias of blocks, up to 25 x 30 m. in cross-section or larger, floating in an enclosing matrix of lime or terrigenous muds. Debris flows vary from lens-like or ribbon channel-fills to irregular beds that are locally adjacent to carbonate platform-basin margins. Some megabreccias are associated with high relief, active tectonism or steep slopes; but others may be the result of pervasive bio-erosion or submarine dissolution of fore-reef carbonates by fresh water springs.
Debris flows have often been misinterpreted as shallow water conglomerates, in situ bioherms, organic mounds and reef talus. Consequently debris flows are probably much more widespread than previously thought. Faunas contained in these deposits include mixed transported shallow-water and in situ deep-water forms.
Changes in base level are probably responsible for many of the above unstable slope deposits. This is because drops in base level will often expose a carbonate basin margin leading to an increase in instability and to slope failure, generating turbidites and megabreccias. Some unstable carbonate slopes and their failure can also result from over production in the shallow-water of the carbonate margin.
Turbidites could have significant source potential because of their origin in shallow water areas of high organic productivity. In addition, their porous facies may act as reservoirs or as conduits for migrating oil. The prolific Cretaceous reservoirs of the Golden Lane field of Mexico are interpreted by some geologists to be a gigantic carbonate breccia deposited by debris flows.
PLATFORM MARGIN
Introduction to Reefs and Organic Buildups
Reefs and organic buildups can form both in shallow normal marine waters where there is a break in slope on the sea floor (figure below), or they may also form landward of this break in slope within the slightly deeper water of platform interiors and epeiric settings. Reefs and buildups have two major forms: either continuous elongate bodies parallel to the depositional strike of the shelf edge or as a series of isolated buildups which may occur on either side of the shelf break.
Reefs
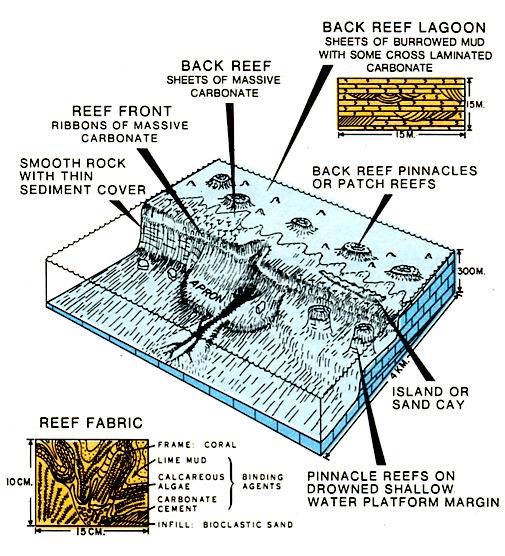
Changes in base level affect the geometries of these buildups. During high stands of sea level the geometries can be both progradational and aggradational, with the general accommodation being driven by the regional subsidence of the depositional setting. During falls in sea level the geometries will generally down step across the previously deposited slope, with the gentler wider slopes forming a substrate to forced regressive wedges of carbonate that step seaward. At the same time updip erosion of the earlier margin can occur, often cutting down to the new sea level. Transgressions can lead to the nucleation of local buildups on local high spots on the downslope portions of the basin margin and the erosion of the updip portions of the earlier margin as it becomes exposed to increasing wave energy.
Barrier Reefs and Mud-Skeletal Banks
Reefs and mud-skeletal buildups are best developed where open marine waters shoal against the basin margin. The slope of the seafloor on which the buildup grows is controlled by antecedent topography, by faulting or by the juxtaposition of active shallow-water accumulation and deeper water basin starvation. Barrier reefs tend to be massive but have associated discontinuous thin beds of sediment. Reef geometry is expressed as thick sheets or ribbons which parallel depositional strike and whose thickness may be related to a particular change in base level. The major sub-environments are the reef frame (the reef crest and reef wall), reef apron, back reef and barrier islands (sand cays). Reefs act as a sediment source to areas both landward and seaward. Major reef contributors through geologic time have included corals, stromatoporoids, calcareous sponges, algae, and rudists. Associated fauna is very diverse. The reef frame is characterized by in situ growth of calcareous organisms interbedded with calcareous sands, silts and muds that form as the result of bio-erosion and episodic storms. The frame is usually massive and cavernous, with void space filled by bladed and fibrous marine cements and by internal sediment that is often perched on or within these cements. Within the reef crest, the skeletal framework may vary from as little as 200/0 of the rock volume to as much as 80%, with a reciprocal distribution of sediment-cement infill.
The reef apron is composed of silt to boulder-sized debris derived from the reef frame and mixed with in situ fore-reef biota. It characteristically has a chaotic texture but may locally exhibit cross-Bedding. Many cited examples of Holocene fore-reef and upper Basin slope deposits contain huge blocks of reef rock that have slumped from the cliff-like fore-reef face. Most precipitous fore-reef slopes are characteristic of Quaternary and Holocene reefs which owe most of their major topographic features to pre-Holocene antecedent topography. Similar fore-reef cliffs occur in the Upper Devonian of the Canning Basin in Australia and portions of the Mesozoic margin of the U. S. East Coast. In general, most pre-Holocene coral reef buildups lack this steep fore-reef cliff, and therefore the associated reef apron sediments have correspondingly less reef core rock rubble. Reef apron sediments may typically be stabilized or encrusted by foraminifera, sponges, or algae. A typical fore-reef toe facies of late Mesozoic and Cenozoic reef buildups is composed of a gravel of irregular red algal nodules. The character to the sediments of the reef apron and foreslope will often have a cyclic variability that is the product of high frequency changes in Base level.
Back reef sediment is formed by both localized patch reef framework that grew as carpets or patch reefs and by skeletal debris transported from the reef crest. The patch reefs tend to be massive and lens-like, while the adjacent back reef sediments are frequently burrowed, widespread, and sheet-like. The sediment varies in grain size from sand to mud. Barrier islands or beaches occur just behind the reef crest and show many of the characteristics of clastic barrier islands. A complex of linear carbonate sand bodies forms them parallel to depositional strike. The seaward margin tends to be smooth but storm wash-over fans and flood-tidal deltas serrate the leeside. Sedimentary structures associated with the islands include cross-laminated carbonate sands from the beach face, lamellar birdseye limestone, algal stromatolites, and storm wash-over layers. Diagenetic changes and cementation may produce beach rock and tepees. As with the reefs described above the character to the sediments of the back reef build ups and sheets will often have a cyclic variability that is the product of high frequency changes in base level.
Nonskeletal carbonate mud and silt banks
Mud-skeletal banks are elongate massive bodies that form both parallel and perpendicular to the seaward edge of the platform margin (left figure). They range from knoll-like mounds of a few square meters to massive linear belts trending for hundreds of kilometers along depositional strike. The thickness of the banks varies from a meter to 100 meters or more. Beds may be thick to massive and range from horizontal to clinoform. Modern carbonate mud banks form in conjunction with sea grasses and green calcareous algae that bind and trap fine sediments derived from breakage in more turbulent water. Sediment in ancient banks of this kind varies from lime mud to fossiliferous sand. The sediment is commonly neomorphosed and may contain cavities filled by internal sediment and cement. The irregular form of the cavities varies according to the position in the bank and the form of roof support. For instance, cavities in the Carboniferous Ivanovia banks that are exposed in the Sacramento Mountains of New Mexico are supported by algal plates and filled by mud and fibrous marine cements. Other Paleozoic mud banks contain similar mud-supported cavities known as stromatactis. These cavities are filled by mud and cement. Again as with settings described above the character to the sediments of the mud banks will often have a cyclic variability that is the product of high frequency changes in base level
Pinnacles, Patch Reefs and Mounds
Pinnacles form during relatively rapid sea level rises when carbonate production only locally keeps pace (figure below). Bottom agitation is not as great over pinnacles, patch reefs, and sediment mounds as it is on shelf-edge reefs, so organisms tend to be different and winnowing and frame building are less important. These structures also differ from shelf-edge reefs in that they are more symmetrical and relatively less oriented with respect to wave and wind directions. The pinnacles and patch reefs are formed by frame builders whereas the mounds are accumulations of lime silt and mud that is trapped by sponges, octocorals, algae, and crinoids.
Pinnacles reefs and sediment buildups are localized landward or seaward of the crest of the Basin margin. They may be localized on highs, formed by previous kharst topography or some other local irregularity, that cause waves to shoal and break, or focus swift tidal currents. Core facies of these bodies are generally massive to thick bedded, while the flank beds have thin, irregular Beds. Changes in texture tend to radiate outward from the buildup core. Seaward buildups commonly contain more porous grain carbonates than the more shelfward mounds, but pore-filling marine cementation occurs more readily in a seaward direction.
As with barrier reef buildups, the pinnacle reefs are characterized by in situ boundstones of calcareous organisms and sediments. The reef frame is massive and cavernous and the voids are filled with sediment and marine cement. These sedimentary features are exquisitely displayed in Silurian pinnacles from the Michigan Basin. Major facies variation is found in buildups that were initiated in deep water and grew upward into shallow water. The basal sediments of these buildups are usually finer grained than the crest. The fauna at the base is usually a pioneer community of low diversity while the fauna of the crest may be a more diverse climax community. Lower contacts are gradational with the platform sediments below the bodies. The pinnacles are commonly overlain sharply by basinal marls and shales similar to those deposited on the deeper parts of the platform and associated with transgressive rises in sea level. Rarely the bodies coalesce upward and are sharply overlain by tidal flat sediments.
Potential Reservoir and Source Rocks
The belt of reef and mud buildups at the depositional surface tends to be a narrow, ribbon-like feature usually less than about l00 m wide. The apron of skeletal sand shed back of the reef may be even narrower, while lagoonal sediments may stretch for tens of miles back of these buildups. In the subsurface all the facies may be quite extensive due to basinward pro-gradation. The reservoir potential of reefs and buildups is widely assumed to be high. However, studies indicate that both primary and secondary cements and internal sediments more often than not plug the porosity of reef bound-stones. Proximal back reef sand deposits can retain significant amounts of primary porosity, especially in reef-tracts where accumulation of skeletal rubble was rapid. Quiet-water carbonates of deeper lagoons tend to end up as muddy sediments (i.e., wackestones and packstones) with relatively low porosities and permeabilities. Fore-reef deposits and the aprons of mud buildups may have somewhat greater reservoir potential; especially if the reef itself is plugged by Carbonate cements and acts as the updip seal in a stratigraphic trap. Some barrier reef deposits have proved to be major hydrocarbon reservoirs, although this facies commonly lacks an immediate updip trap. An excellent example of a giant field in a barrier reef is that of the Oligocene reef complex at Kirkuk, Iraq. Another producing barrier reef of Oligocene age occurs in SE Louisiana and SW Mississippi. A portion of the Devonian Leduc reservoir trend of Western Canada has both the characteristics of a linear mud skeletal margin and barrier reef complex.
Reef tract and linear mud skeletal sediments typically have relatively low source potential. This is in part due to the shallow, turbulent environment but also to the efficient recycling of organic detritus within the reef community trophic structure. Thus little organic debris leaks from the crest community into the apron or into the back margin lagoon.
In contrast to barrier facies, major hydrocarbon discoveries are common in ancient pinnacle reef and mud buildups. Reservoir volumes for pinnacles are likely to be more sharply limited than shelf-edge reservoirs. This is because the porous core facies is typically bounded on all sides by relatively impermeable flank and margin deposits, basinal shales or basinal evaporites related respectively to sea level transgressions or falls. These deposits form the seal but make recharge of reservoir hydrocarbons unlikely. Examples of oil fields in pinnacle buildups are the Silurian of the Michigan Basin and the Devonian of Western Canada. Pinnacles may be associated with fairly rich source rocks at their flanks and within the basinal sediments enclosing them. Organic productivity and preservation in the sedimentary column tends to be high for pinnacles situated on the lower basinal slope but declines updip.
Sand Shoals
Carbonate sand accumulations of reservoir size commonly occur near the seaward edge of banks, platforms, and shelves. Less commonly, they are formed within platform interiors and over topographically high areas within a regionally deep-water setting. Bank-margin sand accumulations may grade over short distances landward or seaward into other carbonate facies.
The development of these bodies requires sand-sized sediment and a means of removing sediment smaller or larger than sand-sized material. These requirements are often met where a change in shelf slope coincides with wave action or strong tidal currents in a zone of high carbonate production. Sand bodies in modern carbonate settings occur in many different forms, nearly all of which have ancient counterparts (left figure). Back-reef sheets, belts and lobes of skeletal sand form along open platform margins where sediment transport is toward the bank. Where small islands exist, the gaps between them may be the site of tidal deltas. Commonly the flood tidal delta is enlarged as a result of storm-created currents. If re-entrants or embayments occur along a margin, tidal and storm-generated currents can generate wide belts of tidal bars. Along windward margins, which are dominated by large islands, skeletal sands generated within the fore-reef environment may be carried seaward to the marginal escarpment. There they may accumulate behind rocky barriers or be carried further seaward into deep water. In contrast to the variety of sand bodies that form along windward margins, leeward open margins are dominated by off-bank sand transport. Here, wide belts or sheets of non-skeletal sands form at the bank edge.
The vertical sequence of deposits found in modern sand shoals often records progressively shallower water deposition because these shallow-water sites provide optimum conditions for carbonate production. As a result platform carbonate sediments usually accumulate at greater rates than the rate of relative subsidence and repeatedly build-up to sea level or above, and thereby form cyclical packages that are each a few meters in thickness. Similar shoaling sequences are recognized in thick ancient carbonate sand deposits. The cyclic character of these shoaling sequences is a response to constant cyclic changes in base level.
Source and Reservoir Potential
Sand shoals do not have good source potential because the environment is oxygenated and highly agitated. Lateral facies equivalents must, therefore, be called upon to act as the source of hydrocarbons trapped in sand shoal reservoirs. In terms of reservoir quality, the carbonate sands have high initial porosities that may be preserved in the subsurface. In addition, because these sand shoals are commonly localized on paleohighs and generally build up to and above sea level, secondary porosity usually develops shortly after deposition. The best documented example of production from sand shoal reservoirs occurs in the Jurassic Smackover Formation of the U. S. Gulf Coast, but other well known grainstones that contain hydrocarbon reservoirs are the San Andres Formation in the Permian Basin and the Arab D Formation which forms the reservoir facies of the worlds largest field, Ghawar! The evaporative seals associated with many of these grain carbonate reservoirs probably owe their existence to a drop in sea level that initiates local isolation and the precipitation of evaporites that then onlap over the reservoirs.
PLATFORM INTERIOR
Epeiric Sea, Lagoon or Bay
This depositional setting, protected by a wide shallow sea, reefs, or mobile carbonate sand barriers, is characterized by continuous wide sheets of poorly-sorted sediments which are commonly extensively burrowed. The sediments either formed in situ or were transported from a seaward barrier by the winnowing action of waves and currents. In the normal marine setting the faunal remains are abundant but not diverse. However, when the setting is some tens of kilometers from the open sea, as in epeiric seas, faunas steadily decrease in species diversity as a response to elevated salinity. At the landward margins of epeiric seas, where salinities are frequently at their highest, the only evidence of life may be subtidal blue-green algal stromatolite heads and mats.
The principal facies in shallow water are clean carbonate sands, muddy skeletal sands, and lime muds, whereas in deeper water marls and shales are common. The sands in shallow water form on stable flats where current energy is sufficient to winnow lime mud but not grains. Grains may be oolitic but are more commonly pellets, grapestones or oncolites. Lime muds form in areas with restricted circulation. Along the land-ward margins of epeiric seas, the muddy sediment is usually dolomitic and stromatolitic. These sediments are well bedded and have wide lateral distribution. In subtidal areas, faunal abundance is low but diversity is high. In contrast, in intertidal areas faunal abundance may be high but diversity is low. Again the cyclic character of these shoaling Sequences is a response to constant cyclic changes in Base level
Tidal Flats
Tidal flat sediments include those forming in the intertidal zone (flooded by daily tides) and the supratidal zone (flooded by wind and spring tides) (figure below). Sediments range from carbonate sands to muds and commonly contain algal stromatolites. Tidal flat sediments occur as widespread sheets that are often dissected by channels. Bedding is thin and even and contacts are sharp; but evaporites show irregular Bedding and may be nodular. Collapse breccias of angular fragments tend to parallel depositional strike and are local.
Traced landward the principal fades belts are: sandy tidal flats, muddy tidal flats, mangrove/algal flats and supratidal flats. The sandy flats, commonly cross-bedded and winnowed, reflect storm wave and current movement. The muddy tidal flats are burrowed and homogenized; Bedding Planes may be irregular, in part a result of the burrowing. Mangroves, algae and other plants bind and trap transported lime mud and may also aid its precipitation. Algae produce a variety of structures in response to desiccation and erosion; lamellar birdseye fabrics and dome heads are the most significant. Tidal creeks rework the tidal flat sediments, developing sandy or muddy point bars. The channel margins may be marked by levees. If the levees are made of lime mud, they generally exhibit a variety of Laminations, mud cracks and Intraclasts. The levees may pond water in the over-bank areas, where sediments are highly burrowed.
Supratidal flat sediments vary according to their climatic setting. For instance, with high salinities and magnesium concentrations Dolomite replaces calcium carbonate and forms a cement. In arid regions gypsum and anhydrite may precipitate directly within the sediment. Simultaneously halite may precipitate locally on the sediment surface, but wind or marine flooding usually removes it.
Reservoir and Source Potential
Tidal flat muds and pelleted sands have low porosities due to dewatering and compaction. However, dolomitization of these deposits forms reservoirs by creating higher porosity and permeability. Tidal flat carbonates commonly are associated with evaporites that act as seals to the reservoirs. Examples of production from tidal flat Sequences include the Ordovician Ellen-burger Formation, the Ordovician Red River of the Williston Basin, Permian Basin carbonates of Texas and the Cretaceous offshore of West Africa. The cyclic character of these tidal flat Sequences is again a response to constant cyclic changes in Base level
Tidal flat carbonates have abundant algal organic matter mixed into them. Although there may be ample opportunity for the organic matter to be oxidized and come in contact with fresh waters, there is evidence that some tidal flat Sequences were deposited quickly enough to maintain a relatively high percentage of organic matter. In addition, there is a growing belief that evaporites may have a sufficiently high organic content to serve as a hydrocarbon source.
TERRESTRIAL
Dunes, Lakes, Cave Deposits, Soils, Fanglomerates
Dunes of carbonate form where there is a source of loose carbonate sand, usually landward of a marine setting or on sand cays (figure below). These fine, well-sorted dune sands exhibit large-scale cross beds that are several meters heigh. The dunes commonly have irregular lower contacts and immediately overlie beach deposits with sharp, upper contacts. Root casts and soil horizons may occur. Dunes form long, linear localized deposits. They have not been recognized very often in subsurface studies, probably because they have been eroded by winds during sea level lows or in a few cases have been misinterpreted.
Lakes may be present in fault-block intermontane basins or on delta plains. Here if terrigenous influx is low, carbonates may accumulate in the lakes. Algal heads, pisolites and beach deposits composed of rock rubble or cross-bedded oolite sand typify Shoreline sediments of lakes. Lake floor deposits consist of alternating light carbonate laminae and dark organic laminae, although terrigenous clays may replace the carbonate muds. The deposits vary from localized thin sheets of individual lake origin to widespread and complex sequences of beds related to stacked lakes. They can be distinguished from
marine deposits by the lack of marine fauna, presence of tufa, and association with other non-marine sediments. Modern examples include the Dead Sea and Great Salt Lake, and ancient examples include the Eocene Green River Formation of the Uinta Basin and Pennsylvanian-Permian Coal Measures of the Illinois and Appalachian Basins.
Cave deposits include travertines, stalagmites, and stalactites, cave pearls and carbonate sands. These carbonates are precipitated during exposure above sea level as waters supersaturated with respect to calcium carbonate are evaporated and carbon dioxide is released. Other deposits that form during breaks in deposition due to exposure are calcareous soils known as caliches. These soils represent a stage of substrate alteration during exposure in semi-arid Mediterranean climates. They are also termed calcrete or nan. Commonly the soil profile is characterized by thin, irregular to continuous layers and pisolites. Laminated soil zones are difficult to distinguish from algal stromatolites. Soil zones are important to recognize in ancient Sequences because they indicate breaks in deposition during which surrounding carbonates may have been exposed to fresh waters and leached.
Fanglomerates are recycled Limestone fragments (lithoclasts) usually found on the down-thrown side of faults in arid regions. The deposits are fan-shaped wedges formed of braided stream and sheet-flow deposits. Braided stream deposits exhibit large-scale festoon cross-Beds and low-angle coarse planar beds that may show fining-upward grading. Channel-fill deposits within the fan are graded beds that fine upwards and show low-angle cross-Beds. The sheet-flow sediments are alternating coarse and fine units with faint grading and some debris-flow boulder layers. Ancient fanglomerates include the Triassic in the Newark Basin and the Tertiary Overton Formation of southern Nevada.
USEFUL REFERENCES
Depositional Environments
Bathurst, RGC, 1975, Carbonate Sediments and their Diagenesis (2nd edn.): Amster dam, Elsevier, 658 p.
Milliman, JD, 1974. Marine Carbonates. Recent sedimentary Carbonates ; pt. 1. Springer, Berlin, Heidelberg, New York, xiv, 375 pp.
Reading, H. G., 1978, Sedimentary Environments and Facies: Elsevier, 557 p.
Scholle, P. A. and Ulmer-Scholle, D. S, 2003, A Color Guide to the Petrography of Carbonate Rocks: AAPG Memoir 77, 474 p
Tucker, ME and Wright, VP, 1990. Carbonate Sedimentology. Blackwell, 482p.
Wilson, J. L., 1975, Carbonate Facies in Geologic Time: New York,Springer-Verlag, 471p.