However, as Kane and Hodgson (2010) note, it is uncommon to identify outcrop analogues of channel-levees, and particularly they find a lack of identifiable internal and external levees. Howver they have found large-scale external levees enveloping channel systems whilst smaller scale internal levees bound individual thalweg channels within the channel-belt. They find this associated with two exhumed channel-levee systems from the Rosario Fm. of Baja California, and the Fort Brown Fm. of South Africa.
Channel-margin levee thickness decreases systematically down-system. They often show a lateral continuity in the facies of the proximal portions of levee facies, but there are often abrupt changes in the lithologies where channel fill and levee sands have an eroded contacts. Conceptual models based on outcrops, borehole images and seismic correlations help with identification of potential reservoir facies (Slatt, 2000).
Slatt et al (2004) record how levees deposits show difference in character related to their proximal to distal position across the levee. proximal levees will tend to have higher net sands, but tend to be thin Bedded with cut and fill features, mud-lined scours, climbing ripples, good connectivity and high angle and variable dipping Beds. The distal levee has lower net sand, thin Bedded, interBedded sand and silt, good continuity, and low angle ripple and the beds dip in a uniformly. The channel margins of levees are more complex, due to slumping. Channel margins are discontinuous, mud-lined, and have variable fluid communication in channel levee reservoirs. The breaching of levees, commonly at channel bends lead to the formation of crevasse-splay complexes. Posamentier and Kolla (2003) record how these features are similar to frontal splays, but smaller in size and commonly are formed by sheet-like turbidites. Channel levee and overbank examples from the Gulf of Mexico exhibit variable oil-water contacts across the reservoir.
Overbank areas
Slumps
Where ever the slope of a basin margin becomes too steep to support the load of the sediment that is accumulating there, slumping of sedimentary material occurs. This can happen on unprecedented scales, as for instance in the Beaufort Sea and the submarine margin of the Nile Delta. Sediment that collected on the over-steepened upper and lower slope is commonly deformed by creep or more rapid downslope movement. Evidence of this movement is expressed in the intra-formational deformation of the sediment or sometimes by huge slump scars. Slumping is also common in mini basins connected and dissected by submarine canyons and valleys. As the channels downcut into the margins and floors of these basins, their margins oversteepen and fail, depositing slumped material over the sheet sands that form the overbank fill marginal to the channels.
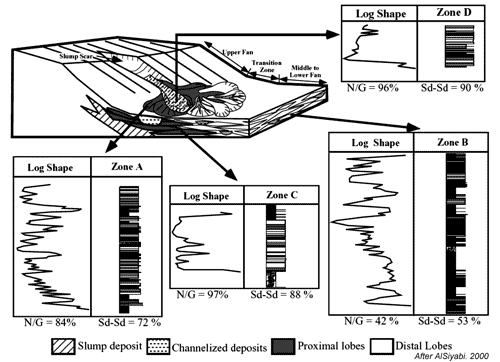
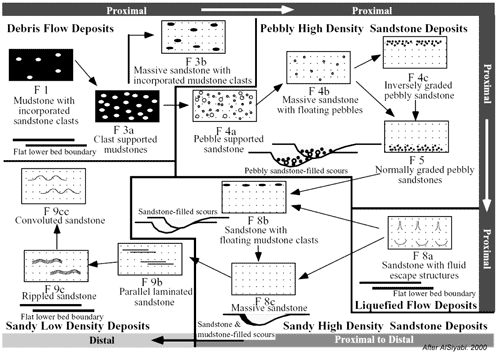
Debris flows (link to further details)
Debris-flow deposits form many of the same features of that turbidite sands express. These range from low-sinuosity channel fills, narrow elongate lobes, and sheets and are characterized seismically by contorted, chaotic, low-amplitude reflection patterns (Posamentier and Kolla, 2003). These deposits commonly accumualte on striated or grooved pavements that can be up to tens of kilometers long, 15 m deep, and 25 m wide. Posamentier and Kolla (2003) indicate that where the flows are unconfined, divergent striation patterns probaby reflect the flow direction and behaviour. Debris- flow deposits can extend at least as far basinward as turbidites, and individual debris-flow units can reach 80 m in thickness and commonly are marked by steep edges (Posamentier and Kolla, 2003). Transparent to chaotic seismic reflection character suggest that these deposits are mud-rich.
Marine shales
see section on deepwater sediments
Surfaces
Allen (1983) established, using fluviatile sediments as an example, that there at least four kinds of boundaries: concordant non-erosional (normal Bedding) ; discorcordant non-erosional ; concordant erosional; and disconcordant erosional contacts.
Click on highlighted Deepwater Gallery for access to a complete index to images, maps, diagrams and photographs of deepwater geology including the geology of Co Clare or access this gallery using the pull down menu on the header bar above.
References Cited
Allen, J. R. L. 1983, "Studies in fluviatile sedimentation: bars, bar complexes and sandstone sheets (low sinuosity braided streams) in the Brownstonews (L. Devonian), Welsh Borders". Sedimentary Geology, 33, 237-293.
Al-Siyabi, H. A., 2000, Anatomy of a type II turbidite depositional system: Upper Jackfork Group, Degray Lake area, Arkansas, in A. H. Bouma and C. G. Stone, eds., Fine-grained turbidite systems, AAPG Memoir 72/SEPM Special Publication 68, p. 245–262.
Beaubouef, R.T., Rossen, C., Zelt, F., Sullivan, M.D., Mohrig, D., and Jennette, D.C., 2000, Deep-water sandstones, Brushy Canyon formation West Texas: Field Guide for AAPG Hedberg Field Research Conference, April 15-20, 1999, AAPG Continuing Education Course Note Series #40, 48p.
Csato, I., C. G. St. C. Kendall, 2001, "Modeling of stratigraphic architectural patterns in extensional settings – Toward a conceptual model", Computers and Geosciences.
DeVay, J. C., D. Risch, E. Scott, C. Thomas, 2000, A Mississippi-sourced, middle miocene (M4), finegrained abyssal plain fan comples, northeastern Gulf of Mexico, in A. H. Bouma and C. G. Stone, eds., Fine-grained turbidite systems, AAPG Memoir 72/SEPM Special Publication 68, p. 109–118.
Gardner M.H. and J.M. Borer, 2000, Submarine Channel Architecture Along a Slope to basin Profile, Brushy Canyon formation, West Texas, in Fine-Grained turbidite Systems/edited by A. H. Bouma and C. G. Stone. AAPG Memoir 72, SEPM Special Publication No. 68., p195-214
Hampton, Bret D., Thomas V. Wilson, and Robert Crookbain, 2006, “From Euphoria to Reality: One Operator's Experience with Reservoir Performance in the Deepwater Gulf of Mexico” Connectivity and Performance of Deepwater Reservoirs (SEPM), AAPG Annual Convention, April 9-12, 2006 Technical Program
Kane IA, and Hodgson DM, 2011, Sedimentological criteria to differentiate submarine channel levee subenvironments: exhumed examples from the Rosario Fm. (Upper Cretaceous) of Baja California, Mexico, and the Fort Brown Fm. (Permian), Karoo Basin, S. Africa. Marine and Petroleum Geology vol 28 pp 807-823
Miall, A.D., 1985, Architectural-element analysis: A new method of facies analysis applied to fluvial deposits: Earth-Science Reviews, v. 22, p. 261–308
Peakall, J., W. D. McCaffrey, B. C. Kneller, C. E. Stelting, T. R. McHargue, and W. J. Schweller, 2000, A process model for the evolution of submarine fan channels: implications for sedimentary architecture, in A. H. Bouma and C. G. Stone, eds., Fine-grained turbidite systems, AAPG Memoir 72 /SEPM Special Publication 68, p. 73–88
Posamentier, H.W., Allen, G.P., 1999,. Siliciclastic sequence stratigraphy: concepts and applications. SEPM Concepts in Sedimentology and Paleontology no. 7, 210 p.
Posamentier, Henry W. & Venkatarathnan Kolla, 2003, "Seismic Geomorphology and stratigraphy of depositional elements in Deep-Water Settings", Journal Sedimentary Research, Vol. 73, No. 3, P. 367–388
Slatt, R.M., 2000, Why outcrop characterization of turbidite systems?, in Bouma, A.H., C. Stelting, and C.G. Stone (eds.), Fine-grained turbidite systems, Amer. Assoc. Petrol. Geol. Mem. 72/Soc. Sediment. Geology Spec. Publ. 68, p. 181-186
Slatt, Roger, James Forgotson, Jr., and Azzeldeen A. Saleh 2004, Petroleum geology of the the deepwateer Jackfork Group and Atoka formation with a primer on the petrokeum geology of deepwater depositionla systems, http://www.pttc.org/solutions/sol_2004/539.pdf
Sprague,A. R., P. E. Patterson, R.E. Hill, C.R. Jones, K. M. Campion, J.C. Van Wagoner, M. D. Sullivan, D.K. Larue, H.R. Feldman, T.M. Demko, R.W. Wellner, J.K. Geslin1 (2002), "The Physical stratigraphy of Fluvial strata: A Hierarchical Approach to the Analysis of Genetically Related Stratigraphic Elements for Improved Reservoir Prediction", (Abstract) AAPG Annual Meeting
Steffens, G. S., 1993, Gulf of Mexico deepwater seismic stratigraphy: AAPG Annual Convention Official Program, p. 186.
Stelting, C.E., A.H. Bouma, and C.G. Stone, 2000, Fine-Grained turbidite Systems: Overview, in Fine-Grained turbidite Systems/edited by A. H. Bouma and C. G. Stone. AAPG Memoir 72, SEPM Special Publication No. 68., p1-8
Stow, D.A.V., 1994. "Deep-sea processes of sediment transport and deposition. In: Sediment Transport and Depositional Processes", ed. by K. Pye, Blackwell Sci. Publ. pp 257-293
Stow, Dorrik A.V. and Mayall, Mike, 2000, "Deep-water sedimentary systems: New models for the 21st century", Marine and Petroleum Geology 17, 125-135
Stow, D.A.V. and Mayall, M., editors, 2000. "Deep-water Sedimentary Systems: Thematic Set", Marine and Petroleum Geology, Volume 17, No. 2.
Sprague, A.R.G., Garfield, T.R., Goulding, F.J., Beaubouef, R.T., Sullivan, M.D., Rossen, C., Campion, K.M., Sickafoose, D.K., Abreu, V., Schellpeper, M.E., Jensen, G.N., Jennette, D.C., Pirmez, C., Dixon, B.T., Ying, D., Ardill, J., Mohrig, D.C., Porter, M.L., Farrell, M.E., Mellere, D., 2005. "Integrated Slope Channel Depositional Models: The Key to Successful Prediction of Reservoir Presence and Quality in Offshore West Africa"; CIPM, cuarto EExitep 2005, February 20-23, 2005, Veracruz, Mexico, 1e13 pp.